Research Areas
Mechanical Engineering
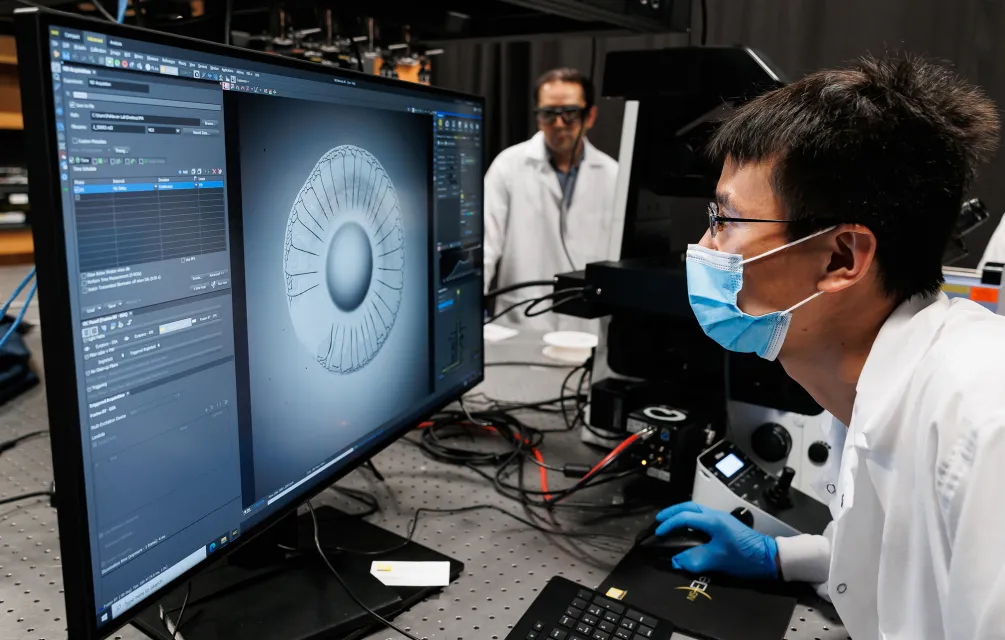
At Yale's Department of Mechanical Engineering, we're advancing scientific discoveries that bridge fundamental physics with transformative solutions. Our research spans from molecular interactions to complex engineered systems, encompassing the principles and phenomena that govern both natural and human-made worlds. Here, students and faculty collaborate far beyond conventional engineering disciplines to address critical challenges, developing innovations that will shape our future. Whether it's creating adaptive robotic systems, investigating complex fluid dynamics, or pioneering new materials, our work catalyzes discoveries that shape tomorrow's technologies.
Fluids, Combustion, & Aerosols
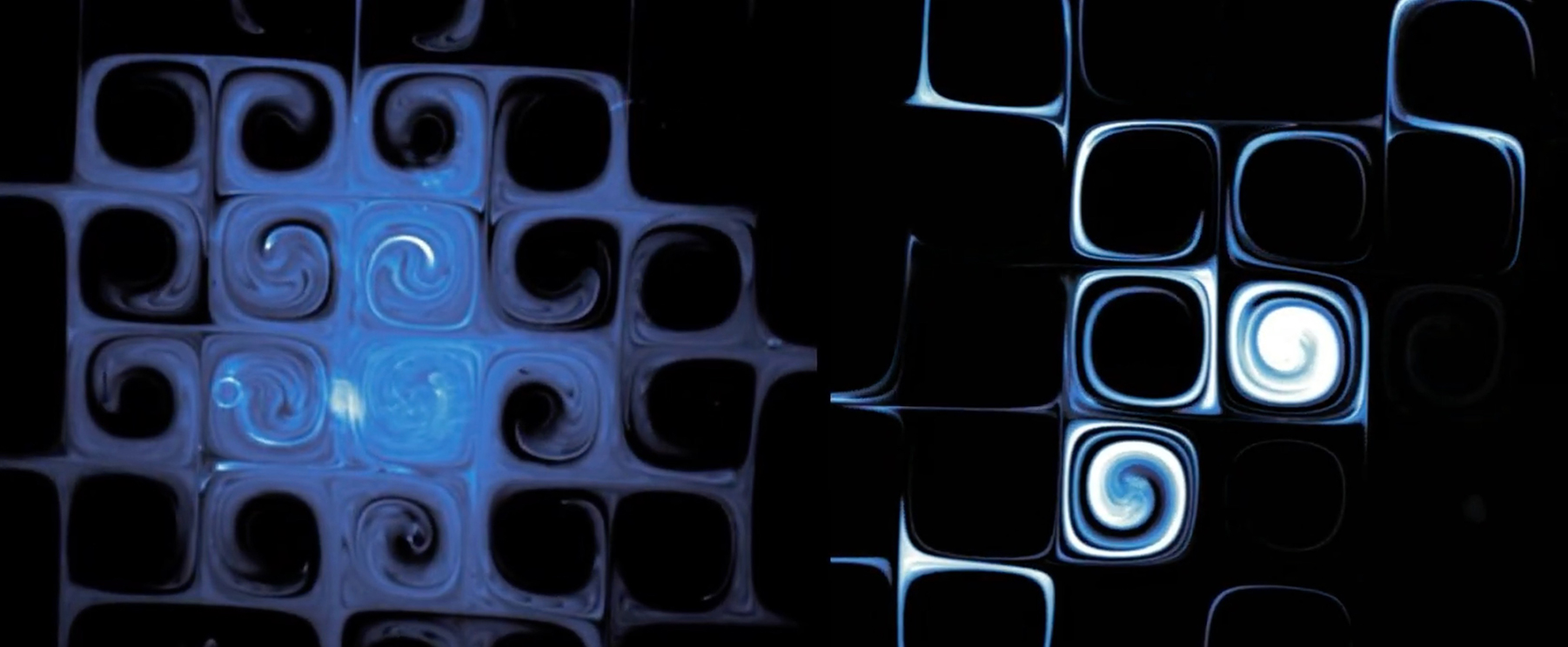
Unlocking Nature's Flow Patterns: From Oceans to Outer Space
Yale Mechanical Engineers are leading groundbreaking research in fluid dynamics, investigating how transport phenomena shape both natural and engineered systems. At the intersection of biology, geosciences, and engineering, researchers study the hydrodynamic interactions among colloids, microplastics, and biological agents in heterogeneous environments. This fundamental research also extends into critical environmental applications, examining how oceanic eddies influence sea ice floe evolution and microplastic transport, while also exploring the complex dynamics of bacteria and biofilms in soil and marine ecosystems.
The department's research in this area combines microfluidic experiments, numerical simulations, and theoretical modeling to understand how fluid flows and structures interact. These investigations reveal unique patterns in vascular networks and microbial communities, while parallel studies examine interfacial flows in confined spaces and phase separation in multicomponent mixtures. Combined, the results challenge classical transport theories by demonstrating how chemical gradients and environmental heterogeneity significantly influence system behavior at multiple length and time scales.
Complementing the work on complex fluids and transport phenomena, Yale researchers are advancing the field through computational modeling of combustion processes and innovative electrospray technologies. Advanced numerical methods enable predictions of pollutant formation in flames, while electrospray techniques facilitate the synthesis of precisely controlled nanoparticles for applications ranging from drug delivery to space propulsion systems.
Associated Faculty

Juan Fernandez de la Mora
Professor of Mechanical Engineering

Alessandro Gomez
Professor of Mechanical Engineering

Amir A. Pahlavan
Assistant Professor of Mechanical Engineering

Bauyrzhan Primkulov
Assistant Professor of Mechanical Engineering

Mitchell Smooke
Strathcona Professor of Mechanical Engineering
Robotics & Control
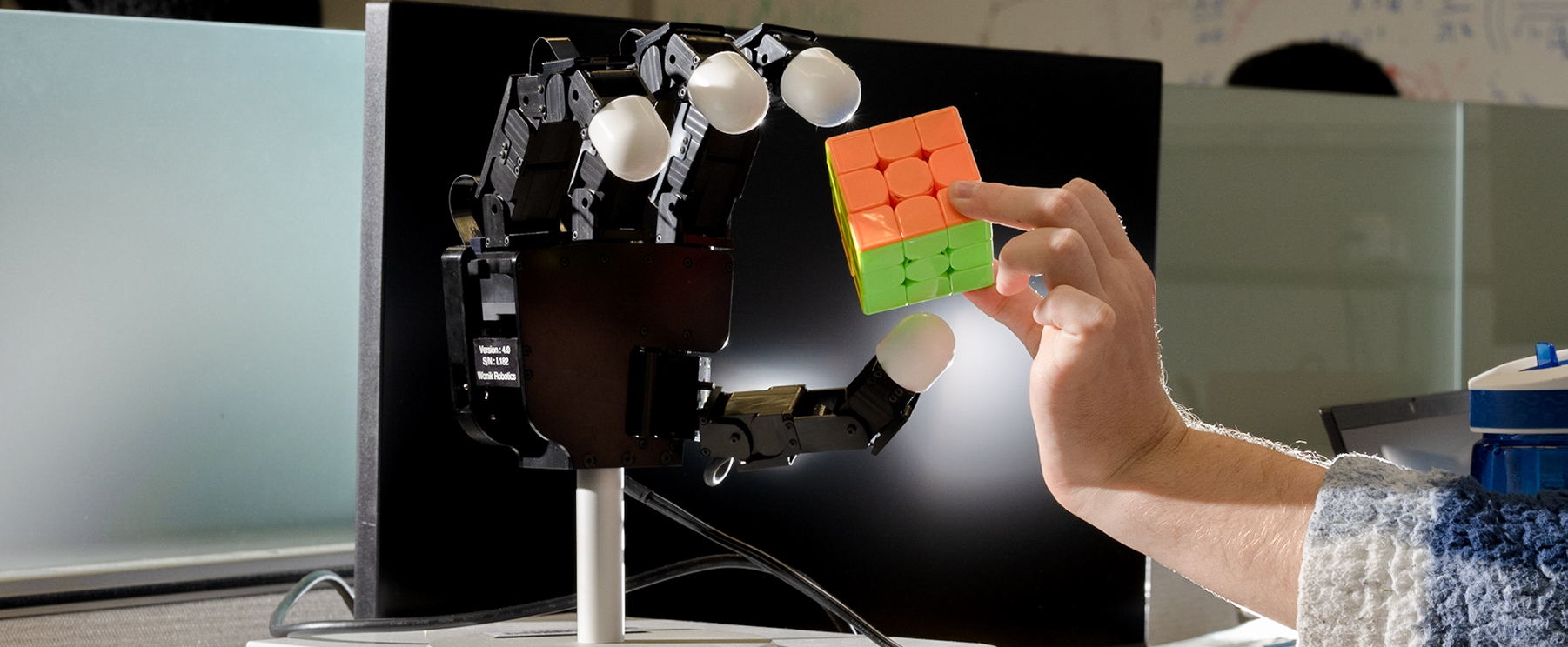
Where Biology Meets Robotics: Creating Adaptive Intelligence
The Department’s robotics and control research bridges the gap between biological systems and robotic capabilities. By studying the fundamental mechanics and control principles of human and animal movement, researchers are developing new insights that inform the design of more capable and adaptive robotic systems. This bio-inspired approach spans a wide range from understanding basic locomotion and dexterous manipulation to creating sophisticated control algorithms that enable robots to learn and adapt to their environments.
The integration of novel materials and innovative control strategies has led to breakthroughs in soft, morphing robots that can adapt their shape and behavior to meet environmental challenges as well as dexterous robotic hands capable of complex manipulation tasks. This work is complemented by research into control approaches that achieve sophisticated behaviors with reduced sensing requirements, making robots more reliable and accessible for real-world applications.
The Department’s researchers deploy a comprehensive approach to robotics research, combining theoretical foundations with machine learning approaches and mechanical design. They are actively developing artificial intelligence systems that enable robots to reason about and learn from their surroundings, while simultaneously creating new mechanical designs inspired by biological systems. This work spans a wide range from fundamental research into the biomechanics of locomotion to applications in prosthetics, environmental conservation, and autonomous systems, with an emphasis on creating robots that can operate effectively in unstructured environments with minimal human intervention.
These research directions are an integral part of Yale Engineering's Robotics for Humanity initiative, which includes partnerships across Yale's campus, fostering innovative solutions that advance robotics technology while addressing challenges in healthcare, environmental conservation, and automation.
Associated Faculty
Mechanics of Materials
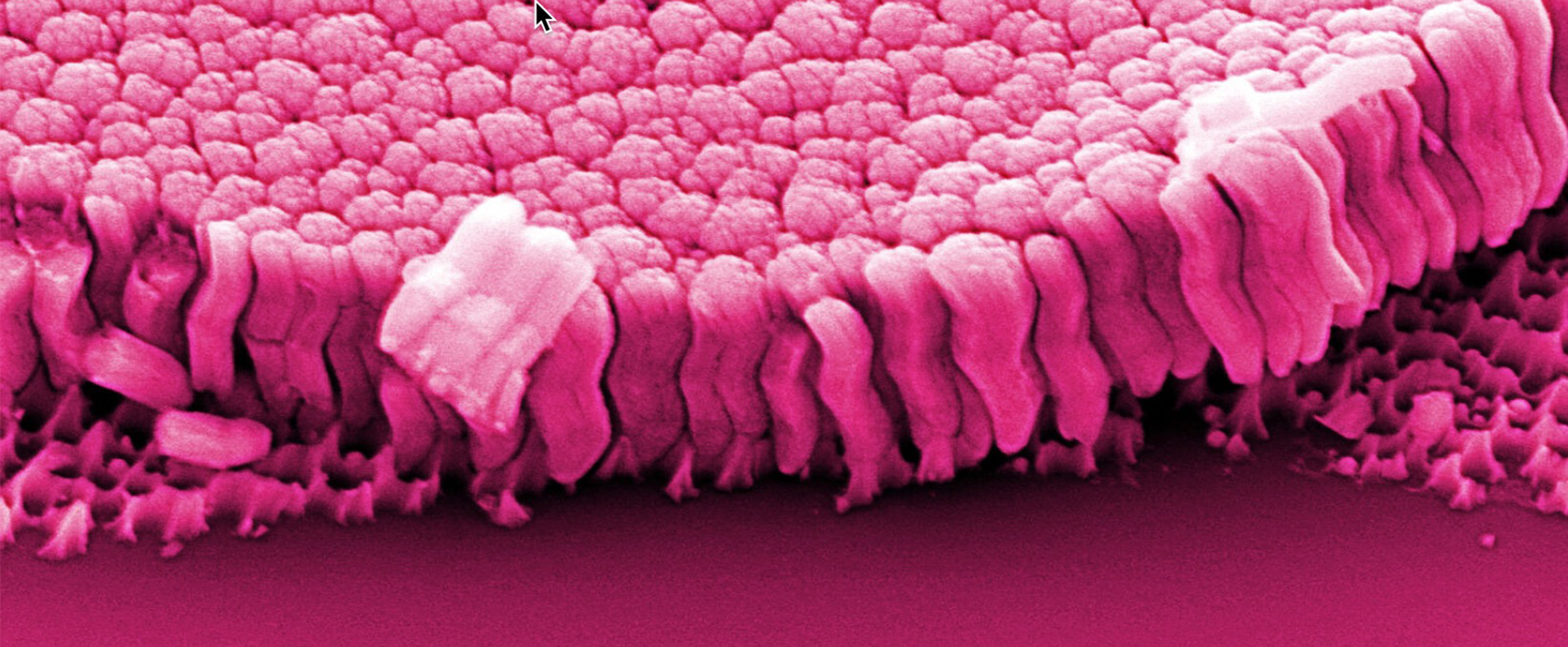
Designing Materials at the Nanoscale
Yale Engineering is pioneering advancements in the mechanics of materials, exploring the intricate relationships between structure, properties, and performance at the atomic and nanoscale. Researchers focus on understanding the fundamental interactions at surfaces and interfaces, using state-of-the-art scanning probe microscopy and other nanoscale imaging techniques. These studies investigate phenomena such as atomic-scale friction, mechanical deformation, and catalytic reactions, uncovering new insights into the behavior of materials like metallic glasses, layered two-dimensional materials, and functional alloys. This work not only advances our scientific understanding of materials but also informs the design of materials with enhanced mechanical and chemical properties.
A key area of investigation is the development of new materials and processing methods to achieve novel functionalities. Metallic glasses, which are amorphous structures with no long range order, are a central focus due to their superior strength and elastic properties compared to traditional crystalline metals. Through techniques such as combinatorial sputtering and nanomolding as well as molecular dynamics simulations, researchers are identifying promising alloy compositions with enhanced functionality and glass-forming ability. These innovations enable applications in energy storage, electronics, and biomedicine, while addressing long-standing challenges in material stability and performance.
Complementing these efforts, theoretical and computational approaches provide critical insights into the atomic-scale mechanisms that determine material behavior. For instance, recent studies have identified nascent atomic-scale defects in amorphous materials that form shear transformation zones and organize into shear bands during applied strain. Other computational studies have identified the key physical features that determine the glass-forming ability of binary and ternary alloys. The integration of experimental techniques with advanced computational modeling allows researchers to design materials with tailored properties, bridging the gap between theoretical predictions and applications. Together, these interdisciplinary efforts are driving innovation and expanding our understanding of the mechanics of materials.
Associated Faculty
Soft Matter & Biomechanics
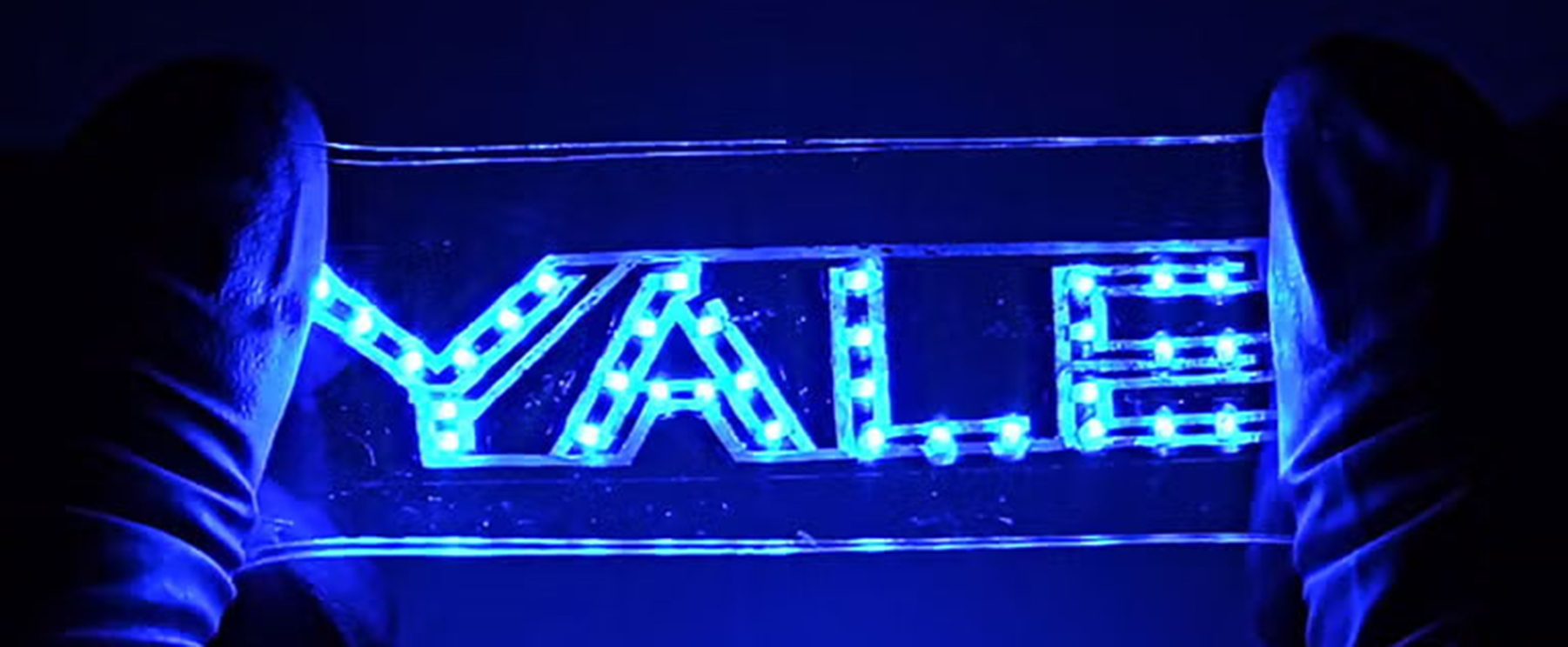
Shape-Shifting Matter: From Living Cells to Adaptive Materials
Yale Mechanical Engineers are advancing the field of soft matter and biomechanics, tackling fundamental scientific challenges while addressing important applications. Our researchers explore the dynamics of soft matter, including collective cell motion, fluid-structure interactions, and adaptable materials. For example, studies of wound healing in epithelial tissues reveal how cells respond to injury and adapt during development, shedding light on mechanisms that could improve recovery outcomes and treatment for congenital disorders. Similarly, advances in computational modeling are redefining our understanding of cancer cell invasion and progression, offering new strategies to inhibit metastasis and improve therapeutic approaches for cancer. Through innovative combinations of machine learning and physics-based models, researchers are also uncovering how groups of cells form complex patterns and structures during tissue development.
This research also includes topics at the intersection of fluid dynamics and materials science. Investigations into bubble pinch-off dynamics and fluid displacement in confined geometries uncover how interactions between liquids, solids, and rough surfaces determine the material properties. These insights are transforming applications from microfluidics and geophysical flows to energy extraction and inkjet printing. By defining the role of surface roughness, researchers have identified novel approaches in understanding the behavior of complex fluids that will pave the way for more efficient designs in geological systems and lab-on-a-chip technologies.
There is also significant synergy between the research areas in Robotics & Control and Soft Matter & Biomechanics. The department's strengths in soft robotics and adaptive materials are pushing the boundaries of what robots can achieve. Innovations in the areas of robotic skins and adaptive materials enable multifunctional and responsive devices that can navigate unpredictable environments with ease. These robotic systems hold promise for applications in search-and-rescue operations, wearable sensing technologies, and space exploration. Together, Yale’s researchers are advancing soft matter and biomechanics through a collaborative, interdisciplinary approach that merges computation, experiments, and theory to address complex materials challenges and unlock new materials functionality.
Associated Faculty

Rebecca Kramer-Bottiglio
John J. Lee Associate Professor of Mechanical Engineering

Yimin Luo
Assistant Professor of Mechanical Engineering

Corey S. O'Hern
Professor of Mechanical Engineering

Amir A. Pahlavan
Assistant Professor of Mechanical Engineering

Bauyrzhan Primkulov
Assistant Professor of Mechanical Engineering

Madhusudhan Venkadesan
Associate Professor of Mechanical Engineering
Theoretical & Applied Mechanics
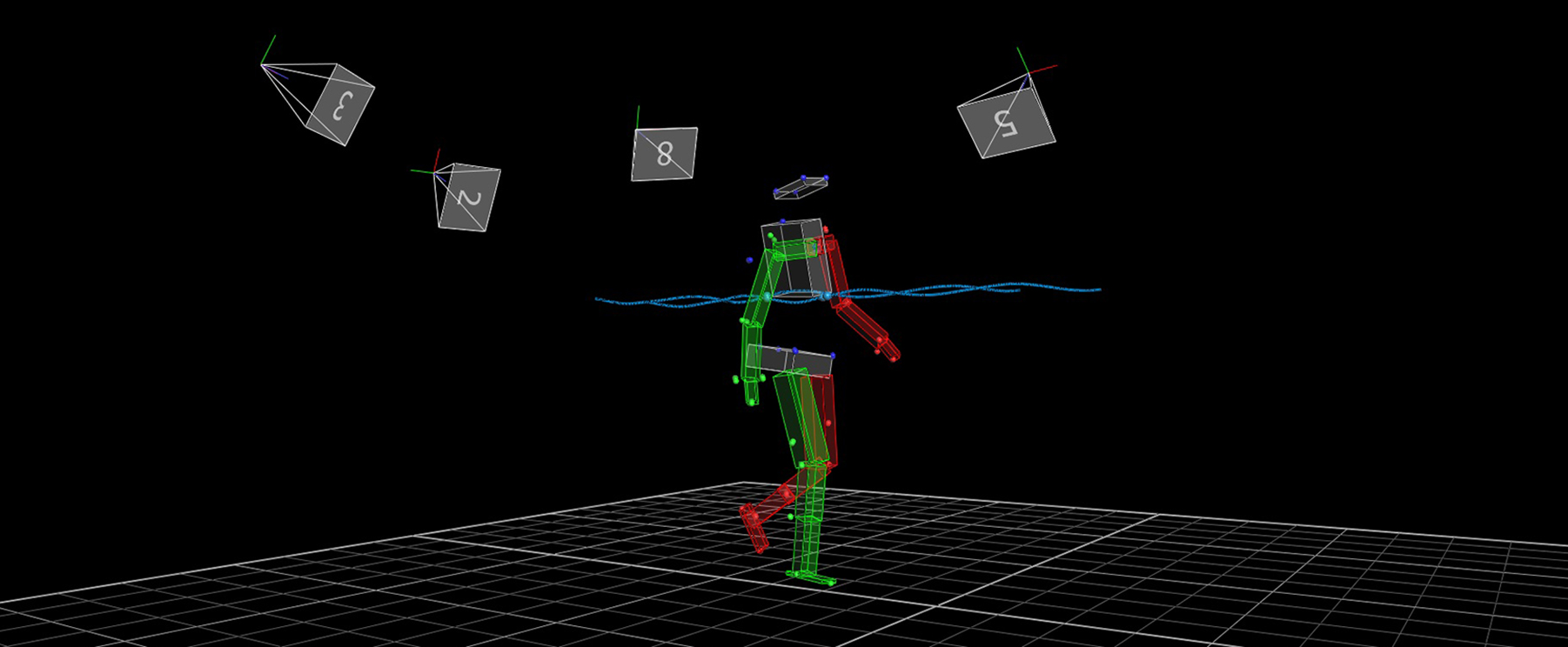
Using Mechanics to Explain Complex Biological and Physical Processes
The Department’s work in theoretical and applied mechanics spans the fundamental physics of nanomechanical and biological systems, from human biomechanics to plant tissue development to the mechanical sensing of atomic interactions. Topics of interest include the understanding of how complex physical properties emerge from basic mechanical principles, e.g., the elastic properties of muscles that enable stable grasping, the intricate physics of human throwing, or the paradoxical development of plant leaves that maintain structural integrity while achieving optimal porosity for photosynthesis. Through mathematical modeling, numerical simulations, and experimental approaches, researchers are uncovering how biological structures balance competing demands, such as speed versus accuracy in human movement, or the structural stability versus functional porosity in plant tissues.
The department's work bridges length scales from atoms to centimeters, from optimizing functionalized nanomechanical sensors for the quantification of site-specific chemical interactions to cellular mechanics and whole-organism function, revealing how evolution has shaped biological structures to achieve remarkable capabilities. These later studies encompass both human systems–including the foot's role as an active suspension system and the muscle properties that enable precise force control–and plant systems, where researchers are decoding the biophysical rules governing tissue development. This research has far-reaching applications, from optimizing catalytically active materials for fuel production to enabling the development of novel biomimetic materials and artificial photosynthetic structures to informing the design of prosthetics and robotic systems, while simultaneously deepening our fundamental understanding of the mechanics of biological and physical systems.
Associated Faculty

Juan Fernandez de la Mora
Professor of Mechanical Engineering

Corey S. O'Hern
Professor of Mechanical Engineering

Amir A. Pahlavan
Assistant Professor of Mechanical Engineering

Bauyrzhan Primkulov
Assistant Professor of Mechanical Engineering

Udo D. Schwarz
Professor of Mechanical Engineering

Mitchell Smooke
Strathcona Professor of Mechanical Engineering

Madhusudhan Venkadesan
Associate Professor of Mechanical Engineering